The “Energy Transition” Delusion A Reality Reset
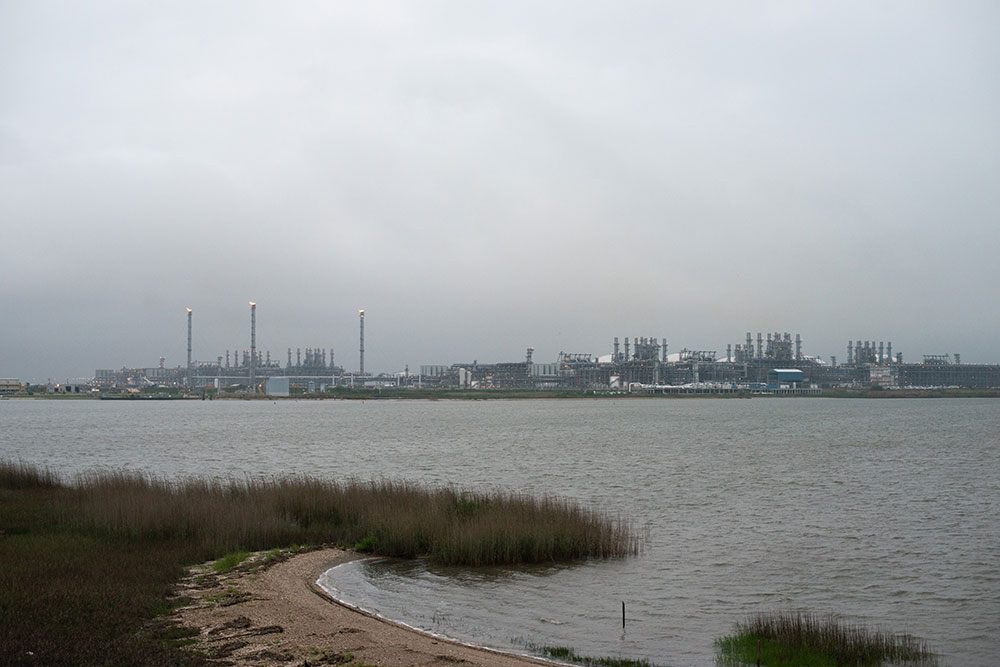
Global economies are facing a potential energy shock—the third such shock of the past half century. Energy costs and security have returned to center stage, as has the realization that the world remains deeply dependent on reliable supplies of petroleum, natural gas, and coal. And all this has arrived during an inflation itself partially the result of higher energy prices that are raising production and transportation costs across industries.
In these circumstances, policymakers are beginning to grasp the enormous difficulty of replacing even a mere 10% share of global hydrocarbons—the share supplied by Russia—never mind the impossibility of trying to replace all of society’s use of hydrocarbons with solar, wind, and battery (SWB) technologies. Two decades of aspirational policies and trillions of dollars in spending, most of it on SWB tech, have not yielded an “energy transition” that eliminates hydrocarbons. Regardless of climate-inspired motivations, it is a dangerous delusion to believe that spending yet more, and more quickly, will do so. The lessons of the recent decade make it clear that SWB technologies cannot be surged in times of need, are neither inherently “clean” nor even independent of hydrocarbons, and are not cheap.
Smart Policy, Straight to You
Don't miss the newsletters from MI and City Journal
The only path to significantly lower energy prices while maintaining vibrant economies—and unlinking them from Russian oil and natural gas—is to radically increase the production of hydrocarbons. The U.S. holds the greatest potential for achieving this outcome, and without government subsidies. On the contrary: increasing the production of these energy sources would generate government revenues, increase U.S. geopolitical soft power, and, in due course, save the world trillions of dollars.
America’s hydrocarbon-centric industries could, if unleashed, replicate the unprecedented growth in oil and natural gas production over the past 15 years. That growth resulted in the U.S. becoming the world’s biggest producer and a major exporter of both. The crucial question now is whether America has the political will to forge an energy path based on the lessons learned and the urgencies of the new geopolitical landscape.
Introduction
Despite ever-escalating rhetoric, an “energy transition” away from society’s dependence on hydrocarbons is not feasible in any meaningful time frame, and it is a dangerous delusion to base policies on the idea that such a transition is possible. Data, not aspirations, show just how critical hydrocarbons are and, in the wake of the Ukraine invasion, the consequences of failing to realize what reality permits. A different understanding of “transition” is required, one that recognizes that new energy sources should be considered additives, not outright replacements, for oil, natural gas, and coal.
Demands that hydrocarbons no longer be used—to generate electricity, heat homes, power factories, or transport people and goods from one place to another—emerge from climate-focused objectives. Observations that they aren’t being replaced and can’t be in any meaningful time frame evoke specious claims of “climate denialism” or the equivalent. But the realities of the physics, engineering, and economics of energy systems are not dependent on any facts or beliefs about climate change.
Meanwhile, current policies and two decades of mandates and spending on a transition have led to escalating energy prices that help fuel the destructive effects of inflation. The price of oil, which powers nearly 97% of all transportation, is on track to reach or exceed half-century highs, and gasoline prices have climbed. The price of natural gas, accounting for 40% of all industrial energy use and one-fourth of global electricity, has soared past a decadal high.[1] Coal prices are also at a decadal high. Coal fuels 40% of global electricity; it is also used to make 70% of all steel and accounts for half its cost of production.[2]
It bears noting that energy prices started soaring, and oil breached $100 a barrel, well before Russia invaded Ukraine in late February.[3] The fallout from that invasion has hardened, not resolved, the battle lines between those advocating for and those skeptical of government policies directed at accelerating an energy transition.
Epitomizing that divide, a tweet from Elon Musk in the immediate weeks following the invasion acknowledged that “we need to increase oil & gas output immediately.”[4] By contrast, the president of the European Commission had announced: “We are doubling down on renewables. This will increase Europe’s strategic independence on energy.”[5] The International Energy Agency (IEA) and the Biden administration are on board with this approach. Indeed, Congress has recently enacted legislation to expand subsidies and spend hundreds of billions of dollars more in that pursuit.
Yet as the Ukraine war dragged on, a policy bifurcation emerged. On one hand, Europe is expanding commitments to SWB technologies, even including bans on conventional car sales within the next decade or so.[6] On the other hand, the European Union (EU) simultaneously reanimated access to conventional energy supplies from Saudi Arabia to Egypt; Germany built its first ever liquefied natural gas (LNG) import terminals; France and Germany refired coal power plants.[7] Behind these contradictory responses is the fact that Russia is one of the world’s three largest producers and exporters of petroleum and natural gas. EU nations depend on Russia for about 25% of their oil and 40% of their natural gas.
The loss of a major share, never mind all, of Russia’s energy supplies would trigger the third and greatest global energy shock since the invention of the computer. Given that hydrocarbons are essential for contemporary society, the consequences of shortfalls or bans would be severe. The first two global energy shocks in the modern world—the 1973 Arab oil embargo and the 1979 Iranian revolution—triggered oil price increases of 200% and 400%, respectively, and touched off global recessions.[8] Each one had long-lasting impacts on policies, government spending, and geopolitics.
This time, there’s potential for even greater harm because, unlike the previous two oil-centric shocks, the Russia-Ukraine crisis also involves natural gas at a scale comparable to the oil at risk. While oil keeps everything moving, natural gas keeps the lights on and is an irreplaceable chemical feedstock that keeps manufacturing supply chains humming. Russian gas provides both the heat and feedstock for one of the world’s largest chemical hubs, Germany. Loss of more than half the supply there would lead to shutdowns and thus shortages and price spikes in key global materials, not to mention massive layoffs.[9]
While oil and gasoline prices continue to rise, we have yet to experience (as of this writing) a loss of supply or rise in prices comparable to the two previous energy shocks. In such a case, JPMorgan analysts recently noted, oil could hit $380 a barrel.[10] The real possibility of an outcome such as that is what motivates political scrambling, publicly and behind the scenes, for alternative supplies of hydrocarbons.
Against this backdrop, consider that years of hypertrophied rhetoric and trillions of dollars of spending and subsidies on a transition have not significantly changed the energy landscape, nor have they altered the long-standing geopolitical tensions inherent in supplying fuels critical for survival. Civilization still depends on hydrocarbons for 84% of all energy, a mere two percentage points lower than two decades ago. Solar and wind technologies today supply barely 5% of global energy. Electric vehicles still offset less than 0.5% of world oil demand.
Naiveté about energy realities has robbed the U.S. and Europe of important soft power options to counter Russian ambitions, i.e., the kind of geopolitical leverage that Russia is currently wielding against Europe and the U.S. regarding fears of the economic and social consequences of shortfalls in critical energy supplies. In the near term, options to Russia’s exports are limited. But doubling down on the energy policies of the past couple of decades won’t significantly impact the need for hydrocarbons. Instead, it’s a formula for more problems in the future, both geopolitical and economic. One of those problems is inflation.
The main trigger for inflation is a rising supply of money sloshing through the economy, typically caused by a government’s massive deficit spending—“printing money.” In the present circumstances, this spending was motivated by the economic destruction of the pandemic lockdown policies combined with the current U.S. administration’s ambitious expansion of social programs. Federal spending in both absolute and relative terms hasn’t been this high since World War II.
But the amount of money circulating through the economy is not the whole story. In normal times, energy typically accounts for just under 10% of the cost of most products and services.[11] Doubling the cost of energy will have an inflationary impact on the average final price tag for all products and services.[12] Impacts are obviously more severe for the most energy-intensive activities such as farming, flying aircraft, or fabricating polysilicon for solar cells.
The U.S. inflation rate surpassed a 40-year high this past October.[13] The last time the Federal Reserve, under Paul Volcker, pushed an aggressive increase in interest rates was in reaction to more than a decade of inflation-inducing federal policies that, combined with the 1979 oil price crisis, triggered a severe recession.[14]
Today’s episode of rising energy costs emerged from a combination of self-inflicted wounds and unanticipated forces damaging global fuel infrastructures. It started with government policies and political pressure that have been, for decades, hostile to expanding the production of conventional energy. From the North Sea to America’s offshore domains—and across most European nations and U.S. states—policymakers actively opposed and even banned the expansion of hydrocarbon infrastructures. Then pandemic lockdowns wreaked havoc on the global economy and energy supply chains. The year 2020 saw the biggest annual decline in global energy demand in nearly a century.[15] The combination of the decline in demand and uncertainty about how long this would last disrupted operations, exploration and expansion plans, and the livelihoods of the energy sector’s skilled workforce. Finally, when the world was already well on the way to $100 oil, the invasion of Ukraine rattled energy markets about possible supply interruptions from Russia, one of the world’s three biggest producers.
Economists ignore the current trends by assuming rising energy prices are necessarily only a short-term episodic influence on broader inflation.[16] As far as supplies of commodities including energy are concerned, the belief is that history has shown that generally, the cure for high prices is, high prices. But will the current price escalation be a brief episode, or will we face a policy-driven era of persistently high prices? If the latter occurs, then the world will enter another period similar to what Federal Reserve historians call the Great Inflation that lasted from 1965 to 1982, when “the inflation being caused by the rising price of oil was largely beyond the control of monetary policy.”[17]
Policymakers ignore at their political peril the importance of energy costs. Gallup’s long-running tracking poll about what people volunteer as the “most important problem” finds that the economy and inflation top the list by a huge margin. The number two issue was “government/poor leadership.” The Russia “situation” was halfway down the top 10. Climate change didn’t make the top 10.[18] (That doesn’t mean citizens “deny” the idea of climate change. Indeed, most citizens say “yes” if prompted with a specific question about whether they believe anthropogenic climate change is happening.[19])
Given the destructive reminders about the importance of low-cost energy—and the brutal lessons, now visible again, about the geopolitics of energy supplies—it is past time to reset energy policies based on reality, not wishful thinking.
Immutable Energy Realities
One can begin with a reality that cannot be blinked away: energy is needed for everything that is fabricated, grown, operated, or moved. It’s easy to ignore the benefits of cheap energy when it’s cheap, but not so much when it isn’t. Consider, to take one example, that more than half the recent rise in wheat prices arose directly from far higher costs for the natural gas used to make fertilizer.[20] Consider as well that digital devices and hardware—the most complex products ever produced at scale—require, on average, about 1,000 times more energy to fabricate, pound for pound, than the products that dominated the 20th century.
Historically, the energy costs of manufacturing a product roughly tracked the weight of the thing produced. A refrigerator weighs about 200 times more than a hair dryer and takes nearly 100 times more energy to fabricate. But it takes nearly as much energy to make one smartphone as it does one refrigerator, even though the latter weighs 1,000 times more.[21] The world produces nearly 10 times more smartphones a year than refrigerators. Thus, the global fabrication of smartphones now uses 15% as much energy as does the entire automotive industry, even though a car weighs 10,000 times more than a smartphone.[22] The global Cloud, society’s newest and biggest infrastructure, uses twice as much electricity as the entire nation of Japan.[23] And then, of course, there are all the other common, vital needs for energy, from heating and cooling homes to producing food and delivering freight.
Advocates of a carbon-free world underestimate not only how much energy the world already uses, but how much more energy the world will yet demand. There are more people, more wealth, and more kinds of technologies and services than existed when President John F. Kennedy faced the Cuban Missile Crisis in 1962, and 60 years later, global energy consumption has risen more than 300%. In the future, there will be yet more innovations and more people, many of whom will be more prosperous and want what others already have, from better medical care to cars and vacations. In America, there are nearly as many vehicles as people, while in most of the world, fewer than 1 in 20 people have a car.[24] More than 80% of the world population has yet to take a single flight.[25] Drug manufacturing is far more energy-intensive than fabricating cars or aircraft, and hospitals use 250% more energy per square foot than commercial buildings.[26]
In terms of energy supply, my earlier reports detailed the challenges, indeed the impossibility, of an energy transition directed at eliminating the use of hydrocarbons for what society needs today, never mind in the foreseeable future.[27] And as I and others have also pointed out, this is not to question whether electric vehicles (EVs) and solar/wind technologies are now dramatically better than in years past. Of course, they are—and that will stimulate, even without mandates and subsidies, greater use of those technologies. But policies to decarbonize energy have demoted the primacy of the three key metrics that have long dominated mankind’s access to energy: low costs, high reliability, and geopolitical security. While numerous new means to deliver energy to civilization have emerged throughout history, they have not led to transitions eliminating the use of previous means. Instead, new means have served as additions to society’s options, shifting the relative importance of each while expanding prosperity.
The core challenge for energy transition goals today arises not from policies or political philosophy but from the physics of energy and technology. Put simply: policy aspirations and soaring language cannot change the existence or nature of, for example, the laws of thermodynamics. The consequences of the underlying physics of energy are visible in five key realities, summarized below: real-world costs, the velocities of big systems, the use of materials for building all machines, the locus of key materials suppliers, and the inflationary impact of forcing markets to adopt minerals-intensive energy systems.
Costs
Claims that wind, solar, and EVs have reached cost parity with traditional energy sources or modes of transportation are not based on evidence. Even before the latest period of rising energy prices, Germany and Britain—both further down the grid transition path than the U.S.—have seen average electricity rates rise 60%–110% over the past two decades.[28] The same pattern is visible in Australia and Canada.[29] It’s also apparent in U.S. states and regions where mandates have resulted in grids with a higher share of wind/solar energy. In general, overall U.S. residential electricity costs rose over the past 20 years.[30] But those rates should have declined because of the collapse in the cost of natural gas and coal—the two energy sources that, together, supplied nearly 70% of electricity in that period.[31] Instead, rates have been pushed higher thanks to elevated spending on the otherwise unneeded infrastructure required to transmit wind/solar-generated electricity, as well as the increased costs to keep lights on during “droughts” of wind and sun that come from also keeping conventional power plants available (like having an extra, fully fueled car parked and ready to go) in effect by spending on two grids.
None of the above accounts for the costs hidden as taxpayer-funded subsidies that were intended to make alternative energy cheaper. Added up over the past two decades, the cumulative subsidies across the world for biofuels, wind, and solar approach about $5 trillion,[32] all of that to supply roughly 5% of global energy.
Whether it’s to cool a home, heat steel, or power a data center, the eternal engineering challenge has always been to find the lowest-cost way to make energy available when it’s needed to meet inherently variable demands, especially in the face of inevitable challenges from nature’s attacks as well as supply chain and machine failures. Oil, natural gas, coal, and even wood and water are easy to store in very large volumes at very low cost, but not so electricity. Hence, grid-scale electric availability has been made possible by using electricity-producing machines (turbines) that can be turned on when needed, fueled by large quantities of primary energy sources (such as natural gas, coal, and flowing water) that are easily and inexpensively stored. Such metrics characterize, for now, more than 80% of U.S. electricity production and more than 90% of transportation.[33] The U.S., on average, has about one to two months’ worth of national demand in storage for each kind of hydrocarbon.[34] Such enormous quantities are possible because it costs less than $1 a barrel per month to store oil or the energy equivalent of natural gas.[35] Storing coal is even cheaper. Thus, over the past century, engineers achieved the feat of building a nation-spanning group of electricity grids that powers nearly everything, anytime, while still consuming less than 3% of the GDP.
Storing electricity itself—the output from solar/wind machines—remains extremely expensive despite the vaunted battery revolution. Lithium batteries, a Nobel-winning invention, are some 400% better than lead-acid batteries in terms of energy stored per unit of weight (which is critical for vehicles). And the costs for lithium batteries have declined more than 10-fold in the past two decades. Even so, it costs at least $30 to store the energy equivalent of one barrel of oil using lithium batteries.[36] That alone explains why, regardless of mandates and subsidies, batteries aren’t a solution at grid scales for days, never mind weeks, of storage.
Even though wind/solar machines don’t have fuel costs and have lower maintenance costs than combustion machines, grid-scale battery costs would have to drop at least 20-fold to match the reliability economics of conventional dispatchable power.[37] There is no physics, never mind engineering or economies of scale, that points to such a possibility. Indeed, battery costs are now rising (explained shortly). To put this bluntly: at today’s and likely future prices, building enough batteries to store 12 hours of electricity for the U.S. would cost about $1.5 trillion, and that scale of storage would still leave the nation regularly third-world dark. The alternative? Build about $100 billion worth of conventional hydrocarbon-based backup that can keep lights on for days and weeks when needed, not just hours. The California Public Utilities Commission has correctly observed, in a diplomatic understatement, that the transition path it has charted will mean that “energy bills will become less affordable over time.”[38]
The lithium battery is what made it possible to build useful EVs. Even so, today it still costs at least 50%–70% more to buy an EV instead of a comparable standard car. Purchase price aside, conventional wisdom has it that consumer reluctance to embrace EVs also arises from so-called range anxiety, which, it is argued, is solved with lots of charging stations. Yet most EVs already have a range equivalent to gasoline-powered cars, 200–400 miles. The issue isn’t range; it’s the time it takes to refuel a battery.
A standard gasoline station pump can fill a fuel tank in about five minutes. It takes about 10 hours to charge an EV with the standard Level 2 charger used for homes and at many public locations.[39] While a so-called supercharger can drop that to 30–40 minutes, both the supercharger hardware and the longer fueling times have dramatic cost implications. A longer time to fuel an EV means that a filling station will need many more fueling “pumps” to support the same number of customers at peak times. And that increased number not only requires far more (expensive) land but also comes with a per-unit capital cost of a supercharger roughly double the cost of a gasoline pump.[40]
The combination of these factors translates into 10 to 20 times the costs of the fueling infrastructure to provide the same functional utility. This doesn’t include the incremental costs to upgrade local electrical distribution infrastructures to handle the higher power levels needed for fast charging. A single supercharger requires electrical infrastructure equivalent to that needed for 10 homes.
Velocities
Russia’s invasion of Ukraine has forced European policymakers to face up to the difficulty of making rapid changes to very large systems. The EU plan announced in May would attempt to substantially reduce Russian imports over the coming five years.[41] Over that period, one can imagine unpleasant actions Russia could take as a reaction, some of which have already happened, including throttling back energy deliveries now or even surreptitious sabotage of alternative suppliers for Europe.[42] The plan focuses on the need to find other places to buy hydrocarbons. It raises, by five percentage points, the previous goal for the renewable share of all energy by 2030. And it also subsidizes and mandates conservation efforts and rationing, intended to achieve a decline in Europe’s overall energy use.
Yet for perspective on the global goal of rapidly replacing all hydrocarbons, as well as Europe’s goals, consider what history shows about the velocity of building global energy infrastructures.
The IEA’s net zero energy transition path would require a construction program that could, within 20 years, build out a 15-fold increase over today’s solar/wind hardware.[43] This is a scale equal to (as will be explained shortly) or greater than the expansion of all global energy infrastructures that have taken place over the past 60 years.[44] Setting aside the economics and today’s regulatory challenges, there’s no evidence of construction capabilities that could build so much hardware at a sustained rate nearly triple that of modern history.
The transition vision also entails building infrastructures that require using about a 1,000% greater quantity of material inputs to produce the same quantity of delivered energy as conventional machines.[45] This would be analogous to replacing—not repairing or augmenting—all existing U.S. highways built since 1921 and doing so in about one-third the time it originally took while using 1,000% more materials per road-mile.
Materials
All energy systems require using minerals and materials to build the necessary machines and physical infrastructures. As a seminal IEA analysis observed, an energy transition is a “shift from a fuel-intensive to a material-intensive energy system.”[46] That agency’s estimates for a path that is far short of completely eliminating hydrocarbons show the need to increase the supply of minerals such as lithium, graphite, nickel, and rare earths by 4,200%, 2,500%, 1,900%, and 700%, respectively, by 2040.
These outcomes are not the consequence of design flaws but are inherent in the nature of SWB technologies. Consider, for example, that about 400 pounds more aluminum and about 150 pounds more copper are needed to build each electric car rather than a standard gasoline-powered vehicle.[47] Those are significant increases when manufacturing millions of EVs a year and simultaneously supporting booming demand for other minerals needed to build more wind/ solar machines as well as grid-scale batteries. And this says nothing about the “hidden” use of hydrocarbons in the mining sector that, even before expanding to meet transition demands, already uses about 40% of global industrial energy.[48] (The hidden need for hydrocarbons to mine the materials necessary for EV batteries also means that driving these vehicles doesn’t eliminate as much CO2 as it appears and could even lead to no reductions at all.[49])
A recent World Bank report observed, in an epic understatement, that “ambitious climate action will bring significant demand for minerals.”[50] A similar analysis from ING found IEA’s transition goals would require about half of all current aluminum and copper production and about 80% of global nickel output.[51] Another recent analysis, from IHS/Platts, found that the world will need to more than double global copper mining to meet current transition plans.[52] Fully replacing hydrocarbons using SWB technologies would require a quantity of minerals that exceeds the known global reserves of those minerals.[53]
Meeting such unprecedented mineral demands will require opening far more mines than now exist, and far faster than at any time in history. (The global average time from the qualification of a property to bringing a new mine into operation is 16 years.) Meeting transition goals will require dozens of new mines for each of a dozen classes of minerals, each at the scale of some of the biggest mines in the world today and each requiring tens of billions of dollars of investment.[54] Even if it were feasible, there are still no plans to meet the scale of mineral demands in the time frames contemplated. This means, in short, that regardless of price, policies, and mandates, the world won’t be able to build the machines to meet transition aspirations.
Suppliers
In the complex calculus of energy policies, the decarbonization road map also creates problematic realignments in energy supply chains. Start with the facts that the U.S. today is dependent on imports for 100% of some 17 minerals that are already listed as critical for national and economic security and that, for 28 other critical minerals, U.S. imports account for more than half of existing domestic demand.[55] Factories that assemble batteries or solar hardware in this country would be equivalent to assembling conventional automobiles domestically but importing all the key components and all the fuel.
China’s global market share in refined energy minerals is double the market share that the Organization of the Petroleum Exporting Countries (OPEC) has in oil markets. China is the world’s top producer (40% market share) of aluminum, a metal that belongs on but is usually absent from the list of energy transition minerals. Then there’s the fabrication of polysilicon, the key raw material for solar panels; China has about an 80% market share of all polysilicon supply, itself an energy-intensive material produced on that nation’s low-cost, coal-dominated electricity grid.[56]
Given current events, it’s also notable that Russia produces 6%[57] of the world’s aluminum (four times the U.S. output)[58] and 10% of global nickel (number three in the world) and is one of the top copper producers at about 4% of world supply (roughly equal to that of the U.S.). Chile, the largest copper producer with a 20% market share, has a new socialist president who has promised social justice and environmental reforms in mining.[59] The number two and three producers of copper are Peru and the Congo. The top nickel producer is Indonesia, not generally viewed as having a stable political environment.
The nature of geopolitical dependencies for energy minerals stands in contrast to the state of play for the hydrocarbons that fuel more than 80% of global economies. There are far more suppliers of hydrocarbons, and the dominant players have only a fraction of the market share compared with the markets for energy minerals. And of course, the U.S. is a net exporter of hydrocarbons.
Wind, Solar, and Battery Prices
Today, the energy sector uses less than 15% of the various critical minerals that are also used for other purposes. But if transition goals were achieved, that share rises from 40% to 70% (at least). Just the pursuit of such an increase and shift in commodities usage would lead to higher and more volatile prices. Even in these early days of potential radical increases in demand, lithium prices are already up nearly 1,000% over the past two years, along with copper trading in a range that’s double the long-run history, nickel trading at a five-year high after coming down from recent peaks, and aluminum prices at a 10-year high. Again, this is the case with SWB meeting only a few percentage points of total global energy needs.
Escalating mineral demands further will escalate their prices, which will have two macroeconomic impacts: it will increase the costs of the SWB hardware itself—thereby inflating the costs of already expensive transition policies—and it will increase the costs of other manufactured goods competing for the same minerals. The latter is broadly inflationary, and the former reverses the assumption built into all transition forecasts, i.e., that the SWB hardware inevitably becomes cheaper.
Until now, significant reductions in battery prices have been the happy result of big gains in manufacturing processes, so much so that the raw minerals now dominate, constituting 60%–70% of the cost of a lithium battery. This means that the era of big declines in battery prices is drawing to an end as material costs escalate. Consequently, many forecasts now see rising battery prices.[60] Morgan Stanley expects that EV makers will need to raise prices by 25% because of the lithium price explosion alone.[61] (Vehicle price increases have already been announced by Tesla and BYD, the world’s two biggest EV makers.)
Solar power economics have been similarly transformed. There, too, thanks to improved manufacturing, commodity inputs now constitute about 70% of the overall price of a solar module. But escalated mineral commodity prices have also reversed the long-run declines in solar module costs; prices are up about 50% over the past few years. Materials make up 20% of the overall cost of wind turbines. Current forecasts see wind-turbine prices rising by about 25% over the next year because of higher material costs.
“Rising commodity prices,” an IEA report noted, “have increased the cost of producing solar PV modules, wind turbines and … reversed the cost reduction trend that the industry has seen for more than a decade.”[62] The future costs of SWB technologies will be dominated by what happens in the global mining industry. And, collaterally, the future costs of many other goods will depend on just how far and how fast governments mandate transition policies.
In 2021, a broad analysis by the International Monetary Fund (IMF) concluded that energy transition plans would cause global metal prices to reach historical peaks “for an unprecedented, sustained period of roughly a decade.”[63] The IMF also noted that the “integrated assessment models” for the energy transition “do not include the . . . potential rise in [mineral] costs.” Nor do those models consider the spillover inflationary effect on other uses for minerals.
Metals normally account for a minor share of the cost to manufacture most products. But a recent U.S. Geological Survey (USGS) paper pointed out that doubling aluminum prices, for example, would increase production costs enough to wipe out the entire profit margin for U.S. manufacturers of conventional heavy vehicles, including trucks and buses.[64] The potential for a systemic, long-term rise in mineral prices would be something new. The entire 20th century was a period of a slow decline in average mineral prices,[65] a beneficial trend that began to reverse only about a decade ago[66] and is poised to accelerate if transition plans are pursued.
Finally, in no small irony, oil and gas account for about two-thirds of the energy used in the mining sector with the balance as electricity, most of which is also produced from hydrocarbons, often coal.[67] Energy spending (before the current fuel inflation) accounted for 40% of costs in mining. Transition policies that increase the cost of hydrocarbons will also directly inflate the costs of mining and minerals. (See the Appendix.)
Shale: The “Other” Energy Revolution
The single biggest shift in energy policy goals in modern history has been the pursuit of means to abandon hydrocarbons. On the other hand, the single biggest shift in global energy production in modern history has been the unanticipated increase in hydrocarbons from the shale revolution.
The scale and speed of the technology revolution that unlocked access to vast amounts of oil and natural gas from shale rock by hydraulic fracturing (fracking) and horizontal drilling remain underappreciated. The rise of shale was history’s fastest and greatest addition to the world energy supply. The only comparable expansion occurred in the decade following the opening of Saudi Arabia’s giant Ghawar oil field in the mid-1960s. There is no ignoring the subsequent rise of that nation’s geopolitical and economic power, as well as the associated creation of OPEC.
The pre-pandemic decade of shale expansion added 800% more energy supply to the U.S. than did the (subsidized) expansion of SWB technologies. In fact, the growth in energy supply from shale tech was nearly double that of the entire world’s expansion of solar and wind tech combined. And, as a practical matter, it was the growth of the former that helped enable the rise of the latter by keeping energy cheap, freeing up capital to spend on inherently more expensive SWB mandates, and enabling (until now) low-cost backup.
America’s shale revolution had and still has far-reaching implications—not the least of which is that it led to the U.S. exporting crude oil for the first time in four decades, and at a volume more than double the last peak circa 1960. Policymakers and energy pundits spent a half century wringing their hands about U.S. import dependencies and serially promulgated policies that had little effect on the overall energy infrastructure. Instead, the status quo was reversed by shale drilling and extraction technologies that were developed and financed almost entirely from private activities. The U.S. now exports more crude than five of OPEC’s members.
As with the rise of the great Ghawar field, a key feature of the shale revolution was its velocity of expansion. The shale industry has vaulted from near zero in 2007 to become a $200 billion–plus annual business. By coincidence, the only other industry matching that revenue scale and velocity over that period was the advent and rise of smartphone sales in the United States.
A key feature of the shale industry is the speed at which wells can be drilled. Rather than planning and development that can take years for traditional billion-dollar hydrocarbon projects, each shale well is an individual decision (with decisions distributed among hundreds of companies) involving, typically, one-thousandth the capital, with planning horizons measured in months and drill times in weeks. Notably, the shale story did not emerge from the efforts of “Big Oil,” though many have a hand in that industry now. Instead, the industry emerged from and still involves hundreds of producers and thousands of directly related, specialized service firms. The reemergence of the U.S. as a major exporter of petroleum and natural gas was the primary reason for the roughly 50% drop in the average global price of oil and liquefied natural gas, a worldwide economic benefit that lasted until recently.[68] The net effects of lower prices were to save consumers trillions of dollars and, inversely, to reduce the money sent to producers, especially Russia and OPEC.
The unique speed of the U.S. shale ecosystem, complemented by the equally unique features of America’s capital markets, allows for rapid and resilient responses to wild economic changes, such as three episodes of price collapses in the industry’s short history. The first collapse arrived with the 2008 recession. The second, in 2014, was the result, in large part, of the Saudis and Russians deciding to flood markets, which successfully bankrupted many players in the U.S. shale industry.[69] But shale production expanded dramatically and rapidly after the first two price collapses, thanks as well to improvements in all the relevant technologies. For example, as a fundamental indicator, the efficacy of shale rigs—energy production per rig—improved at an average rate of more than 20% per year over that decade.[70] That means rig productivity doubled every three years.
The third oil price collapse was the result of the 2020 global pandemic lockdowns. These lockdowns led to a steep economic decline and a corresponding drop in energy demand. The consequences of this latest financial blow are still playing out, complicated this time around by an increase in policies hostile to hydrocarbon investment. But the critical geopolitical and economic question for policymakers now is whether a shale resurgence is possible, i.e., whether this country could add as much new energy supply to the world as it had in the previous decade.
How Shale Can Regain Its Mojo
Despite heavy economic and policy headwinds, the U.S. remains the world’s biggest producer of oil and natural gas and is still the biggest exporter of the latter. The political debates over American energy policy are now centered on whether, in effect, to allow the shale industry to fully recover. But given the lessons made obvious by the Russian energy entanglements, the debate should instead be focused on whether the U.S. shale industry could expand yet more—whether America could, say, double oil and natural gas exports.
If U.S. production could expand by as much—or even half as much—as it did over the past decade, that would shift geopolitical alignments and, non-trivially, almost certainly drive down energy prices again. Whether or not such expansion is possible, indeed whether today’s production levels continue, depends on answers to three questions:
- Will technology continue to improve the cost-effectiveness of producing oil and gas from America’s shale fields and, increasingly critical, also with offshore deepwater projects?
- Will capital markets provide the necessary funding, despite active campaigns for divesting from or penalizing firms for such investments?
- Will federal and state governments slow-walk permits or expand outright bans on regulatory permissions needed for infrastructures, from wellheads to pipelines and ports?
Answers to the second and third questions, about money and permissions, emerge from political considerations. There, even if difficult and seemingly unlikely, changes are possible with the proverbial stroke of a pen (no one believed repeal of the decades-old oil export ban would happen and indeed be signed by President Barack Obama). But whether another great expansion of shale production is fundamentally possible is anchored not in legislative ambitions—though legislative permissions matter—but with what technology makes possible.
Higher prices typically lead to increased production, eventually, from somewhere. The issue is always specifically when and from where. The production response to price is determined by the conviction of producers that prices will remain high enough for long enough to recover new capital invested, by whether regulatory permissions will be granted in a timely fashion, and by the available workforce.
We should note that whether the U.S. could radically increase energy production is not a question about physical resources. “Technology unlocks resources,” we pointed out almost exactly 10 years ago, and “North America has total hydrocarbon resources that are some four times greater than those found in the Middle East. The geology of North America is profoundly hydrocarbon-rich.”[71] Thus the anti-hydrocarbon narrative has moved past the flawed idea of limits (“peak oil”) to today’s “keep it in the ground” narrative. And, for the record, we also predicted a decade ago—when discussions were in the thrall of peak oil and it was illegal to export U.S. crude—that the U.S. would become the biggest hydrocarbon producer and should (and would) become an exporter.
One of the flaws in many oil/gas forecasts is in confusing the nature of reserves, a term and a figure narrowly defined by regulatory and securities requirements, as well as the practicalities of near-term business planning. Reserves do not reflect the actual physical quantity of a resource. Official U.S. oil reserves in 1980 were at 30 billion barrels. From 1980 to 2020, the U.S. produced more than 100 billion barrels. Today, official reserves are still listed as about 35 billion barrels. Reality comes from resources that are converted into qualified reserves because businesses, markets, and technologies make progress.
Consider the untapped resources inherent in onshore oil and gas. USGS mapped the existence of shale hydrocarbons about a century ago. Accessing those resources required suitable technological progress, which finally emerged about 15 years ago. But, as the geophysical literature points out, today’s technology releases only 5%–15% of the hydrocarbon molecules locked in the shales.[72] There are knowable pathways for doubling, even tripling, the resources recovered per well.[73] The key to getting them will be the timely perfection of cost-effective technological advances. Relevant to the transition debate: there are no known paths to doubling the energy output of any SWB technology.
Technological progress is also clearly evident by looking at the hardware required per barrel produced from onshore shale fields. When 2020 began, the number of operating oil and gas rigs in the U.S. was only a few percent higher than two decades earlier, yet production was more than double.[74] That productivity gain came from the combination of a learning curve; improved rigs that operated better, faster, and with lower maintenance; the capability to drill multiple wells from a single well-pad; improved drill bit designs; and advances in materials sciences.
Another indicator of technological progress: labor productivity. In the first shale era, from about 2007 to the 2014 price collapse, average labor efficiency, in terms of barrels of oil produced per employee, declined some 30% compared with the pre-shale era. Thus, shale production growth rose in tandem with employment, a trend that is only tolerable in the short term. The key to any progress is to increase labor productivity—greater output with slowly rising or even declining labor. Employment in the oil and gas industry steadily declined from 2015 back to pre-shale era levels while output rose. This translated to record productivity: oil production per employee is now 40% higher than the long-run rate, including before the advent of the shale revolution.[75] That is a textbook definition of productivity, which is the key to economic growth everywhere.
There are also significant untapped offshore resources. Deepwater domains are the only other “fields” whose scale is potentially comparable to U.S. onshore shale. Offshore rigs account today for nearly one-fourth of all the world’s oil. If that domain were a nation, it would be the world’s number one supplier. And experts at Wood Mackenzie have noted that most of the new reserves added to global oil capabilities over the past half-dozen years came from a handful of “high-impact” offshore discoveries.[76] While coastal nations around the world welcome and encourage the development of offshore oil and gas, more than 90% of U.S. offshore domains are entirely off limits to exploration, never mind development.[77] Opening access to just those relatively small areas adjacent to where production is currently permitted (i.e., areas where geophysical data are available) would likely triple America’s overall access to useful oil fields.
The evidence of offshore technology progress is apparent in the speed that challenging deepwater projects are now projected to be brought online—the kind of projects that used to take more than a decade to complete. Shell, for example, expects to have its latest platform in the Gulf of Mexico in production by 2024, just seven years after the discovery and validation of a 500-million-barrel oil field.[78] In the southern Caribbean Sea, Exxon’s offshore Guyana discovery of a 10-billion-barrel field began production in 2020, only a few years after validation.[79] Output from that field is expected to approach 1 million barrels per day by 2027.[80] For context: that’s double Germany’s total oil imports from Russia.[81] All this technology progress, notably, comes from the hard-earned skills of Big Oil companies.
Today, with oil and natural gas prices approaching historic highs, the growth of domestic investment in new production lags far behind the historic levels associated with higher prices, as does, consequently, the growth in oil and gas output.[82] The data also show a recent slowdown in the rate of improvement in shale productivity. There are physical limits, some of which are now being approached, to such things as just how fast holes can be drilled in rock, how many wells can be drilled from a single pad, and the like. Learning curves that push the limits of new techniques always play out in a similar pattern. Thus, the question now is, as it was a decade ago, whether there are foreseeable technology factors offering the possibility of a resurgence in shale production.
The answer is the same as it would be for all mature industries: one looks to technologies that can impact planning, managing logistics for materials and people, and operating relevant machinery. In the shale industry, as well as offshore, these are where revolutions in software and automation are playing out. Practical digital tools are now emerging for the “hard” worlds of machines, manufacturing, and supply chains—rather than where most attention has been focused lately in the “soft” worlds of entertainment, news, and finance. In another recent Manhattan Institute paper and our recent book, we explored in detail the emergence of these new digital capabilities in industries.[83] This transformation is particularly relevant for supply chains and especially energy production.
What’s Changing?
The pandemic lockdowns exposed the vulnerability of industrial supply chains of all kinds; they also damaged those supply chains. Now, despite the winds of recession, there remains a severe shortage of people with the necessary skills across all industries. Enhancing the productive capability of any employee is the practical benefit of using artificial intelligence (AI) tools and automation, with the latter especially in the form of useful robots.
From mining to manufacturing, all processes involve accessing materials and supplies; managers and engineers are deluged with data about multiple factors relevant to myriad operational efficiencies involving sources and quantities of inputs, as well as changes in location or composition of supplies and services. The technologies of digitalization and automation to cope with these data are as relevant for a $10 billion offshore platform as they are for a $10 million onshore shale project. Recognizing patterns in such data is what facilitates better decisions about operating equipment and managing processes, and where the new classes of AI tools can be trained to see and advise. Improving the utility of such previously challenging software tools for frontline workers is the emergence of natural language interfaces. These expand access to systems for people without programming skills or special expertise.
AI tools are increasingly democratized by virtue of the Cloud, which makes supercomputer-powered capabilities available, in real time, on the front lines of the workplace. This promises to reverse the effects of 20th-century software that hollowed out middle-skill work (e.g., drafting, typing, accounting) and moved it onto the desktops of professionals. Now, the combination of AI and the Cloud can “up-skill” the non-expert and move expert decision-making closer to the frontline employees, hollowing out some of the professional-class jobs. As an indicator of its importance, spending on Cloud services, a nonexistent category two decades ago, is now a $250-billion-per-year global business, and one that is increasingly focused on industrial activities.[84]
These trends are bullish for all heavy industries, including the mineral supply chains that are essential for SWB technologies. But, in no small irony, AI and robots can and will also expand hydrocarbon capabilities where adoption is taking place faster. In effect, expansion of the two domains, hydrocarbon versus SWB machines, is a measure of the relative ease of expanding steel-centric hardware (where common, cheap iron ore and coal are the main inputs to steel) for hydrocarbons compared with expanding supplies of copper, nickel, and lithium for SWB tech. The challenge with the latter is easily illustrated by an immutable and consequential fact of geology: at least 5,000% more rock must be dug up and processed to access a ton of copper compared with a ton of iron. While AI and industrial robotics can and will make both domains more productive, they can’t close that gap.
A post-pandemic survey of more than 1,000 professionals in the oil and gas industries found that of “all the cost-efficiency levers, digitalization is the one with the most remaining potential”; nearly 70% of respondents said they had plans to increase such investment, the “highest level ever” in that long-running survey.[85] The ongoing transformation of traditional oil field services has inspired, for example, digital oil field collaborations involving tech companies from Microsoft to Amazon to C3 AI, and traditional energy companies such as Baker Hughes and Shell.[86] Similarly, there are now hundreds of venture-backed tech start-ups focused on every aspect of producing and delivering oil and natural gas.[87] A Barclays forecast sees the global energy-focused digital service industries growing from less than $5 billion now to $150 billion before the decade is over.[88] McKinsey’s year-end 2021 survey found industrial domains a close second to services where the application of AI provided the greatest benefits.[89] (Full disclosure: I am a nonoperating partner in an energy software investment fund.)
The newly practical class of AI-enabled tools is finding applications in complex planning and design processes. Shell has documented one example of a system optimization task that normally involved more than 24 hours of effort yet declined to 20 minutes by using hyperrealistic simulation.[90] Myriad digital-class and automation solutions already exist for optimizing the roles and locations of people and suppliers—predicting where to drill or when to build, real-time control of actual drilling and related tools, drone deliveries and safety monitoring, and robotic pipe handling or robot-driven trucks. Since so much equipment still needs to be operated by humans, AI and automation are now enhancing the training and skill level of heavy equipment operators, emulating the long success of flight simulators for training pilots.[91] It is only recently that simulator technologies have become good enough and cheap enough to use for training on machines that cost a few hundred thousand dollars rather than aircraft that cost millions. More than 1,000 heavy equipment simulators are already in use.[92]
As Tom Blasingame, president of the Society of Petroleum Engineers, recently said, “pervasive digitalisation is the only way I can envision our future activities.”[93] Even so, it will be hard to predict the specific impacts of the digital transformation underway. As leading experts on AI have pointed out, “researchers and policy makers are underequipped to forecast the labor trends resulting from specific cognitive technologies, such as AI.”[94] But only with digitalization will it be possible to achieve cost-effective growth in oil and gas production and enhance the productivity of the available skilled workforce. Rather than automation, as one report put it, “eliminating one out of every five jobs”[95] in the industry, it’s far more likely it will instead allow the five people currently employed to do the work of 10 people.
Escaping the Russian Bear Hug
EU plans to eventually eliminate dependence on Russian energy distill to an “all-of-the-above” strategy. While that means, unsurprisingly, ambitious expansions of SWB tech and enforcing lower energy use, it also means and is dominated by surging investment in hydrocarbons from non-Russian sources. The reason is simple: it is impossible to surge SWB energy outputs in meaningful time frames.
For example, one plan floated by the EU would cost well over $1 trillion, most of which would be spent on more solar and wind hardware, and construction would bypass normal environmental impact assessments—but it would eliminate only a small share of Russian gas imports. And as experts at Rystad Energy observed, those costs exclude subsidies planned for hydrogen, biofuels, and more electric transmission.[96] Rystad also noted that it would “require wartime-like planning, levels of investment, construction, and production,” and still require major importation of oil, natural gas, and coal.[97]
In a nod to reality, Germany will keep older coal- and oil-fired power plants available (and likely its remaining nuclear plants) in case Russia retaliates by cutting off exports of natural gas before alternative supplies can be secured. That boycott would be economically devastating in Europe, a literal lights-out event. Consequently, Germany is building four LNG import terminals at a total cost of $3 billion. Construction began in the second quarter of 2022 and the first terminal will be operational by year-end, the others in early 2023.[98] Those will supply enough fuel annually to match the quantity of electricity produced by $40 billion worth of wind turbines, and the wind turbines would still require natural gas backup much of the year since wind machines operate, on average, one-third as often as gas turbines.
Meanwhile, Russian crude has continued to move into markets through alternative shippers both overtly and via “dark market” traders.[99] Countries including China and India have expanded purchases of Russian energy, enjoying the benefit of discounts. It’s not the first time the world has learned what happens when a country’s exports are sanctioned. Similar efforts directed at Iran and Venezuela saw crude continue to flow via “ghost fleet” tactics, fake registries, spoofing of ship locations, and surreptitious transfers at sea.[100]
Then there are the functional impossibilities of tracking molecules. Russian crude sent to refineries in say, India, is blended with imports from other nations and ends up as diesel fuel sold in Europe.[101] Russian crude even flows to Saudi Arabia at a discount, enabling more Saudi exports at market prices.[102] Perhaps the most telling admission of the difficulty of replacing hydrocarbons, in general, is that European firms are submitting to Russia’s demands to pay in rubles.
Well before the Ukraine invasion, IEA repeatedly noted that signatories to the 2015 Paris Climate Accords have not been meeting their transition promises. The agency nevertheless modeled the outcomes of a more aggressive path. It found that implementing more expensive and expansive transition plans—including prohibitions, for example, on some aspects of car ownership, and mandated building temperature controls—would still only slightly lower global use of hydrocarbons by 2040.[103]
Meanwhile, member nations at the G7 meeting in June 2022 endorsed expanded investments in natural gas and stricter carbon emissions goals. These are likely incommensurable goals in practice, but endorsing both says a lot about the broader transition challenge and the potential emergence of policies based on reality. One bellwether: the EU in July 2022 voted to categorize both nuclear energy and natural gas as official parts of the energy transition, despite vigorous opposition.[104]
Regardless of how the Ukraine crisis plays out, the stability of the world’s energy supply will remain uncertain. In any event, the single most important geopolitical and economic question does not center on how to increase SWB technologies; instead, it’s about who supplies the world with the hydrocarbons that will be needed for many decades into the future.
Time for Energy Realpolitik
This is not the first time that U.S. or European policymakers have attempted to tackle comprehensive changes in energy policy. It began when the U.S. Congress passed the 1975 Energy Policy and Conservation Act (EPAC). EPAC constituted a radical, even panicked, omnibus policy forged in the aftermath of the 1973–74 oil embargo. It also established the framework followed by essentially all energy policies since: avoiding, conserving, or replacing hydrocarbons.
EPAC spawned 50 years of policies that resulted in cumulative spending of well over $700 billion to avoid hydrocarbons (never mind billions more in subsidies, credits, mandates, and state spending).[105] Indeed, Congress passed legislation in 1972 and 1982 banning the exploration or production of oil on about 90% of America’s offshore domains.[106] (Nonetheless, the remaining 10% is responsible for about 15% of all U.S. oil production.)
Today’s Congress and administration are following this dead end—expanding restrictions on drilling in offshore and onshore domains, along with impeding or canceling projects from leases and road accesses on federal lands to pipelines and refineries. Federal agencies from the Securities and Exchange Commission to the Bureau of Land Management—not only the Department of the Interior and the Environmental Protection Agency—have been put to work in an “all of government” push for energy transition and climate objectives.[107] Recently announced revisions to the rules associated with compliance with the National Environmental Policy Act will further increase impediments to infrastructure expansions, in particular those associated with oil and gas.[108] And now, S&P Global has announced a modification to credit ratings for U.S. states based on so-called ESG (environmental, social, and governance) criteria that hold the potential to penalize state governments favoring oil and gas development.[109]
U.S. policymakers are instead proposing increasing federal spending on alternative energy with a kind of “Marshall Plan” modeled on the one proposed by General George C. Marshall after World War II, wherein Congress appropriated and spent about $150 billion (inflation-adjusted) over three years to help rebuild Europe.[110] In June 2022, the EU legislated even more onerous carbon emissions restrictions.[111]
The U.S. oil and gas industry has weathered political storms and price cycles before. But that industry now faces a potent combination of policy and regulatory impediments, higher costs of materials and services, lingering supply chain disruptions, and skilled labor shortages. At least one U.S. shale CEO has said that not even $200 oil would inspire his firm to expand production faster than the current slow growth.[112] And this says nothing about the investment-killing potential of calls for “windfall profits” taxes which, if implemented, would be as destructive and ineffective as they were when tried in 1980.[113]
The world will need yet greater supplies of hydrocarbons to diminish Russian influence as well as fuel economic growth and prosperity. We know where to find and how to produce more hydrocarbons. The non-Russian opportunities are dominated by just three domains: OPEC, deepwater rigs (global and U.S. offshore), and America’s shale fields. If there were ever any doubt that Russia understood the economic and geopolitical threat from the capabilities of U.S. shale producers, one need only look at President Vladimir Putin’s public denunciation of the shale industry a decade ago.[114]
In less than a single decade, the U.S. went from being the world’s biggest importer of petroleum and an importer of natural gas to becoming (for now) the world’s biggest exporter of the latter and one of the biggest exporters of the former. To put this into geopolitical perspective: just the growth in U.S. production over the past decade was greater than Russia’s total supply of oil and gas to Europe. The prospects for U.S. oil and gas firms to expand yet again will require taking the kinds of risks that are invariably associated with adopting new technologies and deploying capital onshore and offshore. Those prospects rely on government actions.
One can imagine—because it could be done—Congress engaging in a reset of energy policy and enacting legislation that would not cost taxpayers money but instead generate profits for American firms and lead to reduced energy prices for consumers. The only way to achieve that would be to encourage and facilitate a radical increase in domestic hydrocarbon production and exports—a shale resurgence along with a similar expansion of deepwater production. It would require a commensurate reset of the regulatory structures that today are not only impediments but hostile to major industrial projects. Washington Post columnist George Will recently wondered: “Can America ‘do big things’ again? Ask the regulators and lawyers.”[115]
The pursuit of an energy realpolitik need not be, nor should it be, framed in terms of replacing ambitions to encourage greater use of SWB technologies. Instead, it should be a recognition that the world needs more “all of the above.”[116] But industries here, and nations everywhere, await meaningful signals from the U.S.—signals that can only come from legislation, not executive orders or rhetoric—that a path is being charted to surge, not shrink, the mighty American hydrocarbon machine.
Appendix: Top 10 Energy Truths
Presented here are 10 truths that show the impossibility of “accelerating” an energy transition that would eliminate the use of hydrocarbons. They also show the consequences of mandating, at a pace faster than would naturally occur, the adoption of wind, solar, and battery technologies.
1. ENERGY TRANSFORMATIONS ARE SLOW
Growth in Global Energy Supplies
The shares of the world’s primary energy sources change very slowly. After at least $5 trillion in spending over the past two decades, hydrocarbons still supply 84% of global energy, down just two percentage points.[117] For context, burning wood still supplies more than five times the amount of global energy than do all the world’s solar panels.[118] Meanwhile, the total demand for hydrocarbons has risen over those 20 years by an amount equal to six times the entire oil output of Saudi Arabia.[119]
2. ECONOMIC GROWTH CREATES DEMAND FOR MORE ENERGY
Per Capita Wealth vs. per Capita Energy Use
As more people around the world become more prosperous, they will want what others already have—everything from better medical care to cars and vacations. The billion people living in wealthy nations use at least 500% more energy per person than the world’s other 6 billion.[120] Wealthy nations have 80 cars per 100 citizens; there are only a few cars per hundred people elsewhere.[121] More than 80% of the world population has yet to take a single air flight.[122]
3. SHALE TECHNOLOGY IS HISTORY’S BIGGEST ENERGY REVOLUTION
Growth of Shale Energy vs. Wind+Solar 2005–20, Compared with Saudi Arabia Oil 1965–80
The scale and velocity of the revolution that unlocked the vast amount of oil and natural gas in America’s shale fields was history’s fastest and greatest addition to global energy supply. The only comparable expansion happened from 1965 to 1980, with the opening of Saudi Arabia’s giant Ghawar oil field, which led to the creation of OPEC and reshaped geopolitics. The pre-pandemic shale expansion added 800% more energy to the U.S. than did the (subsidized) growth of solar and wind combined, and shale energy expanded by nearly double the growth of global solar and wind power production.[123]
4. GREEN ENERGY ISN’T CARBON-FREE
Miles Driven Before an EV Emits Less CO2 than a Diesel Car
Volkswagen (VW) calculates that a diesel car emits less CO2 than an EV for the first 70,000 miles it is driven. How can this be? Fabricating one EV battery entails mining about 250 tons of rock to secure the minerals needed.[124] The energy used in the mining ecosystem—oil, coal, and natural gas—means that one EV has a carbon debt equal to emitting between eight and 20 tons of CO2 before its first mile driven.[125] VW’s calculation is based on the lowest end of that range. The actual debt’s size depends on where minerals come from. Moreover, battery carbon debts will be greater in the future because mineral ore grades are decreasing, requiring the mining of more rock, which means using more energy.[126] Realistic scenarios could lead to EVs emitting more total CO2 than conventional cars over their lifetime.[127]
5. ENERGY TECH CAN’T EMULATE THE DIGITAL TECH PERFORMANCE CURVE
Lithium Battery Performance Progress
Lithium battery performance—energy stored per pound—has tripled since its introduction, though progress has slowed in the past decade.[128] The target performance for batteries—and even the (still pre-commercial) aspiration for super-density lithium-metal chemistry—still doesn’t come close to matching gasoline. An EV has a massive half-ton battery to match the range of about a dozen gallons of gasoline weighing 85 pounds. Advocates claim that “energy tech” can conquer that gap, often analogizing with the progress of digital tech—computing’s exponential growth—but such a comparison isn’t just flawed; it’s impossible in the physics of energy. If lithium chemistry could emulate digital progress since 1990, an EV today would have a battery the size of a single flashlight C cell, not one weighing 1,000 pounds.
6. ENERGY TRANSITION HARDWARE RADICALLY INCREASES THE DEMAND FOR MINERALS
Mineral Demands for Solar, Wind, and EV to Replace Hydrocarbon Machines
All energy systems require minerals for building machines. Using wind/solar/batteries to provide the same power as machines that burn oil, natural gas, or coal requires increasing the use of minerals such as copper, by about 300%, and others such as lithium, graphite, nickel, and rare earths by 4,200%, 2,500%, 1,900%, and 700%, respectively.[129] Similar increases are needed to build EVs instead of conventional cars. (Replacing hydrocarbons with solar and wind facilities also uses at least 1,000% more steel, concrete, and glass to produce the same amount of energy.) The aggregate impact on mineral demands is far greater than both existing and planned global mining capacities.
7. ENERGY TRANSITION POLICIES ARE INFLATIONARY
Energy Sector Share of Mineral Demands for All Purposes
The energy sector today uses only about 10%–20% of the total global production of various minerals. But IEA transition goals—even ones far short of eliminating hydrocarbons—would raise that to a 50%–70% share, or more, including using half of all of today’s aluminum supply.[130] Such unprecedented demand would drive mineral prices higher, raising the costs to build products in every market using those minerals (e.g., appliances, houses, vehicles, electronics), including the alternative energy machines themselves. If pursued, IMF economists predict that metal prices would reach historical peaks “for an unprecedented, sustained period of roughly a decade.”[131]
8. GREEN ENERGY ISN’T CHEAP
Costs of Wind, Solar, and Battery Hardware
Minerals and metal commodities constitute 60%–70% of the cost to produce a solar module (such as a rooftop panel) and a lithium battery, more than 20% of the cost of a wind turbine.[132] The long-run declines in the final price of all three have slowed and reversed. IEA points out that future commodity price escalations could “eat up” any anticipated reductions in manufacturing costs for transition hardware.[133] EV makers have already raised sales prices because of higher materials costs.[134] The future cost of transition hardware will be dominated by technologies and policies in the mining sector.
9. CHINA IS THE OPEC OF GREEN ENERGY MINERALS
Sources of Key Energy Transition Minerals
China’s overall market share of energy transition minerals is double OPEC’s share of oil markets.[135] China is also the top producer of aluminum, critical for transition machines, with a 40% market share. The U.S. is dependent on imports for 100% of some 17 critical minerals, and, for 28 others, net imports account for more than half of domestic demand.[136] Russia produces 10% of global nickel production (number 3 in the world) and 6% of the world’s aluminum (four times the U.S. output).[137] Chile, the largest copper producer—Peru and the Congo are numbers two and three—has a 20% market share and a new socialist president who has promised environmental “reforms” in mining.[138]
10. MARKETS AND CONSUMERS WANT RELIABLY CHEAP ENERGY
Share of Economies Consumed by Acquiring Fuel and Food
Providing billions of people with cheap and reliable energy has been one of the greatest achievements in history. Moving fuel into the twilight of economies has freed up capital for pursuits in health, environmental protections, comforts, leisure, and entertainments. That progress has been entirely a consequence of the inherent physics and economics advantages of the great expansion in the extraction and use of hydrocarbons.
About the Author
Mark P. Mills is a Manhattan Institute senior fellow, a faculty fellow at Northwestern University’s engineering school, and a partner in Montrose Lane, an energy-tech venture fund. He is author of the book The Cloud Revolution: How the Convergence of New Technologies Will Unleash the Next Economic Boom and a Roaring 2020s (2021), and previously: Digital Cathedrals (2020), Work in The Age of Robots (2018), and The Bottomless Well (2006). He served as chairman and CTO of ICx Technologies, helping to take it public in 2007. Mills served in the Reagan White House Science Office, and before that he was an experimental physicist and development engineer in microprocessors and fiber optics.
Endnotes
Are you interested in supporting the Manhattan Institute’s public-interest research and journalism? As a 501(c)(3) nonprofit, donations in support of MI and its scholars’ work are fully tax-deductible as provided by law (EIN #13-2912529).